雷サージからの監視カメラの保護
今日の社会では、監視カメラは至る場所に存在します。近所で朝のジョギングをしているときも、子供を学校に送り迎えしているときも、通勤しているときも、防犯カメラに監視されている可能性があります。米国人は毎週約238回も監視カメラに映っています。防犯カメラは設置が簡単で安全であるという理由から、私有地と公共施設の両方で普及が進んでいます。セットアップが完了すると、カメラはすぐに画像とビデオの記録を開始し、許可されたユーザーがスマートフォンなどのデバイスを介してインターネット経由でリアルタイムのビデオフィードにアクセスできるようになります。
Surveillance cameras are typically outdoor and expected to operate flawlessly for many years with minimal upkeep. These cameras must maintain accurate performance despite the challenging outdoor conditions and remain resilient against threats like electrostatic discharge (ESD), electrical fast transient (EFT) and surges due to lightning strikes and electrical switching. Ensuring the enduring dependability of surveillance cameras necessitates a comprehensive understanding of relevant standards and the thoughtful incorporation of transient protection devices that align with those safety standards.
IEC Standards for ESD, EFT and Surge Protection
The International Electrotechnical Commission (IEC), a global organization dedicated to fostering international collaboration in the realm of standardization for electrical and electronic industries, has formulated transient immunity standards that now serve as essential benchmarks for manufacturers. These standards encompass the three primary guidelines aimed at addressing diverse transient surges:
1. IEC 61000-4-2: This standard focuses on ESD in electronic applications, considering the system-level impact within an end-user environment. Click here for a detailed overview of this standard.
2. IEC 61000-4-4: This standard assesses the equipment's ability to withstand recurring electrical fast transients and bursts.
3. IEC 61000-4-5: This standard addresses the most severe transient conditions caused by lightning strikes and switching surges on both power and data lines.
In this blog, we will delve into the IEC 61000-4-5 standard and explore how to safeguard electrical components used in security cameras for data/signal lines against lightning and surge transients per this standard.
IEC 61000-4-5
IEC 61000-4-5 outlines the procedures and methodologies for conducting surge immunity testing of any equipment under test (EUT). We can begin by defining what a surge is. A surge is a short-lived, high-energy voltage and current spike, sometimes triggered by electrical switching or lightning. Unlike ESD and EFT events, surges persist longer and contain higher energy levels.
IEC 61000-4-5 addresses transient conditions on power and data/signal lines. Switching transients can arise from various factors, including power system switches, alterations in power distribution loads, short-circuit faults, or instances of distributed ground caused by electrical arcing. Lightning-related transients can occur due to direct strikes on outdoor equipment, induced voltage and current from electromagnetic fields resulting from indirect lightning strikes or ground disturbances from lightning coupled to the entire grounding system.
Surge Generator or Combination Waveform Generator (CWG)
The IEC 61000-4-5 standard defines a test setup to simulate a surge or transient scenario, a transient entry point to the EUT, and a set of installation conditions. The main idea is to create waveforms that closely resemble real-world surge events. The transient is defined as a surge generator or Combination Waveform Generator (CWG) producing a given waveform and having a specified open circuit voltage and source impedance. There are two combination waveforms defined by the IEC 61000-4-5:
• 1.2/50µs combination wave (1.2/50µs into open circuit and 8/20µs into short circuit)
• 10/700µs combination wave (10/700µs into open circuit and 5/320µs into short circuit)
The 10/700µs combination wave generator is used to test ports intended for connection to outdoor symmetrical communication lines. In this blog, we will discuss only 1.2/50µs combination wave generators. A simplified schematic of the surge generator for a 1.2/50µs combination wave is shown in Figure 1.
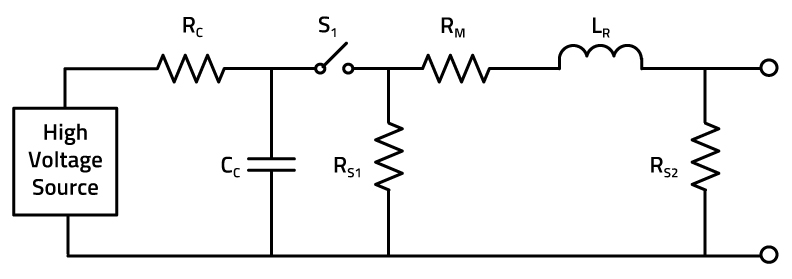
Figure 1. Simplified circuit diagram of the combination waveform generator (1.2/50 µS & 8/20 µS)
The CWG, as per the IEC 61000-4-5 standard, uses a test setup that simulates the characteristics of a surge as both a voltage waveform and a current waveform. In Figure 1, the high-voltage source charges the capacitor CC, via a resistor RC. When the capacitor Cc is charged, the switch S1 delivers the energy into the network created by RS1, RS2, RM and LR. RS1 and RS2 are the pulse duration shaping resistors, RM is the impedance matching resistor and LR is the rise time shaping inductor. Component values are chosen intentionally to ensure they produce a defined voltage surge into an open circuit and a defined surge current into a short circuit. The two waveforms exhibit variations in both their rise times and durations. The voltage waveform shown in Figure 2 (a) has a rise time of 1.2µs and a half-length of 50µs. The current waveform has a rise time of 8µs and a half-length of 20µs. The test setup delivers a 1.2µs/50µs voltage waveform when the outputs are open, and it provides an 8µs/20µs current waveform when the outputs are short.
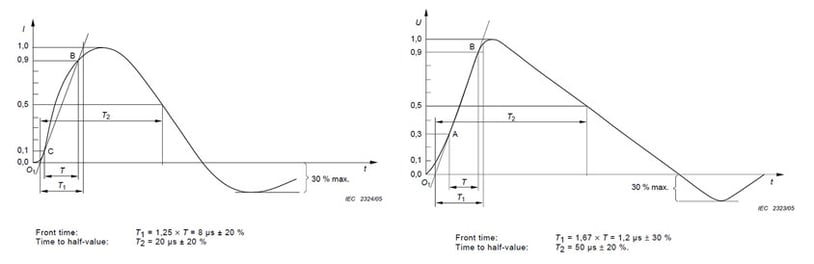
(a) (b)
Figure 2. Waveform of (a) open-circuit voltage (1.2/50µs) (b) short-circuit current (8/20µs) (Source)
The critical factor in generating these waveforms lies in the choice of components for the circuit depicted in Figure 1. To simplify matters, one convention that has been adopted is the determination of the generator's effective output impedance. This definition is based on the ratio between the highest open-circuit output voltage and the highest short-circuit current produced by the generator. In the case of CWG, this ratio indicates an effective output impedance of 2Ω. Essentially, this 2Ω signifies the impedance of the cable or any other connections within the system. The peak voltage typically ranges from 0.5kV to 4kV. Table 1 shows the relationship between the peak short-circuit current and peak open-circuit voltage.
Table 1: Relation between peak short circuit current and open circuit voltage
Coupling/Decoupling Network
The IEC 61000-4-5 standard outlines various coupling/decoupling networks, which are tools designed to couple or transfer the voltage surge generated by the CWG to the EUT while isolating or decoupling it from the facility power supply. The objective is to safeguard the network of the EUT by mitigating the impact of surges and excessive voltage on other connected devices. When coupling the CWG to the EUT, the output impedance can be much higher than 2Ω in several circumstances. The determination of this factor relies on the specific applications, whether it involves a signal line or a power supply network, as well as the location of the surge impact. IEC 61000-4-5 provides guidelines about the selection of external coupling networks. The reason for adding an external resistor is to reduce the energy in the applied surge. When conducting surge tests on signal or communication lines (unshielded, unsymmetrical interconnection lines) a coupling network consists of a 40Ω external resistor as shown in Figure 3. This configuration yields a source impedance of 42Ω, which comprises the sum of the external resistor (REXT=40Ω) and the inherent impedance (ROUT=2Ω). In Figure 3, the CD denotes coupling devices that guarantee adequate insulation between the interconnection lines and the CWG while facilitating the efficient transmission of the surge impulse. Any coupling device, whether it be capacitors or gas discharge tubes (GDT), which can fulfill the requirements for coupling and insulation, is suitable for use.
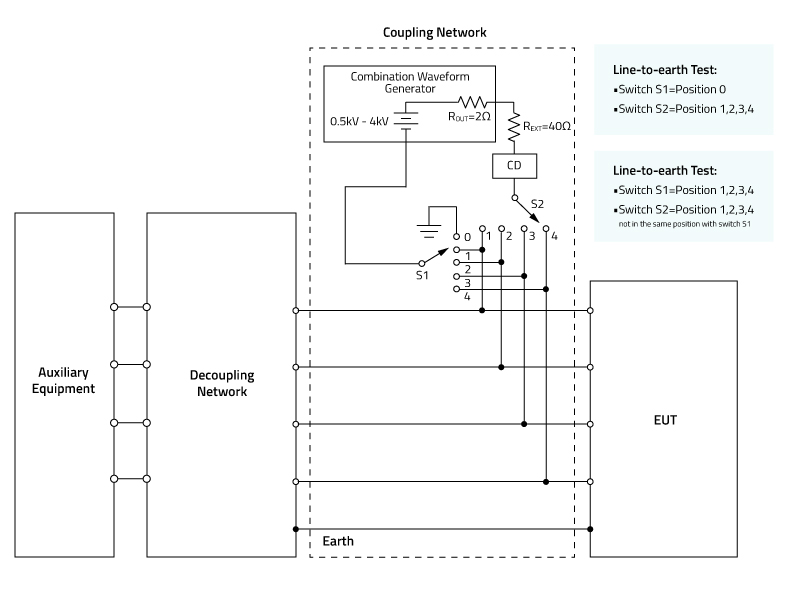
Figure 3. Test setup for coupling network for unshielded unsymmetrical interconnection lines:
line-to-line and line-to-earth coupling
When a directly coupled surge event affects the input of any EUT with severe current, the components within the circuit board connected to the signal lines experience an inductively coupled attenuated surge current. To illustrate, if the CWG is charged to 500V, the coupling network will generate a peak current of 500V/2Ω, resulting in a substantial 250A surge that the EUT will experience at its power input. However, the signal line encounters a peak current of 500V/42Ω, resulting in a 12A surge. Therefore, the source impedance of 42Ω plays a significant role in attenuating the peak current while keeping the surge voltage constant.
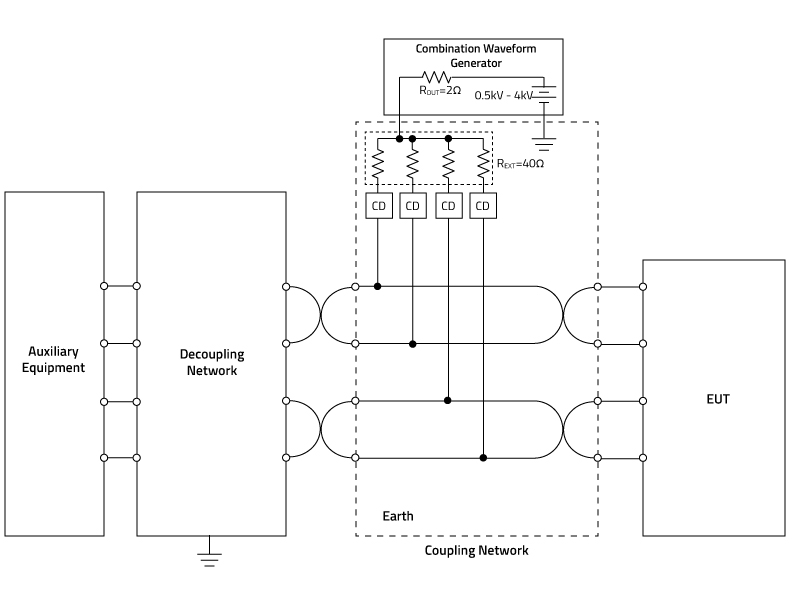
Figure 4. Test setup for coupling network for unshielded symmetrical interconnection lines: line-to-earth coupling
Figure 4 illustrates the test arrangement for coupling and decoupling networks used to subject unshielded, symmetrical interconnection lines to surges. When coupling with symmetrical interconnection lines or twisted pairs, the coupling occurs in a common mode, meaning it affects all lines in relation to the ground. The energy transfer from the surge generator to the EUT is considered to be a constant, irrespective of the number of lines in the cable, and is equivalent to a coupling impedance of approximately 40Ω. This equivalent coupling impedance is distributed among the lines within the cable, and that is why the value of the coupling resistor employed on each line is a multiple of 40Ω. For example, each resistor in Figure 4 will be 160Ω, so the total equivalent REXT is 40Ω.
Transient Stress Levels and Surge Currents
Having gained an understanding of the CWG circuit and the coupling/decoupling network for signal line test configurations, the last piece of information we seek regarding the IEC 61000-4-5 standard pertains to the transient stress levels and surge currents. Installation classes define transient stress levels for each entry point into any system. The IEC 61000-4-5 standard delineates various classes based on the equipment's installation location, with each class having an associated peak voltage requirement. The definition of the six installation classes is shown in Table 2. The equipment generally requires transient surge protection if it belongs to installation class 1 or confronts at least a 500V surge and above.
Class | 環境 | Voltage Level |
0 |
Well protected environment
|
25V |
1 |
Partially protected environment
|
500V |
2 |
An electrical environment where the cables are well-separated
|
1kV |
3 |
An electrical environment where the power and signal cables run in parallel
|
2kV |
4 |
Multi-wire cables for both electronic and electrical circuits
|
4kV |
5 |
Electrical environment where pieces of equipment are connected to telecommunications cables and overhead power lines
(low-densely populated areas)
|
Test Level 4 |
Table 2: Installation classes and voltage levels
Tables 3a and 3b summarizes various threat levels on signal lines based on the installation class and coupling methods. It presents voltage stress values using the 1.2 x 50 µs waveform. For selecting suppression elements, it's more beneficial to consider the short-circuit current values. These short-circuit current stress levels are defined using the 8 x 20µs waveform.
Installation Class |
Unsymmetrical operated circuits/lines |
|||||||
External Port |
Internal Port |
|||||||
Coupling mode: Line-to-line |
Coupling mode: Line-to-earth |
Coupling mode: Line-to-line |
Coupling mode: Line-to-earth |
|||||
Voltage |
Current |
Voltage |
Current |
Voltage |
Current |
Voltage |
Current |
|
0 |
NA |
NA |
NA |
NA |
NA |
NA |
NA |
NA |
1 |
NA |
NA |
NA |
NA |
NA |
NA |
0.5kV |
12A |
2 |
NA |
NA |
NA |
NA |
0.5kV |
12A |
1kV |
24A |
3 |
NA |
NA |
NA |
NA |
1kV |
24A |
2kV |
48A |
4 |
2kV |
48A |
4kV |
95A |
2kV |
48A |
4kV |
95A |
5 |
2kV |
48A |
4kV |
95A |
2kV |
48A |
4kV |
95A |
Installation Class |
Symmetrical operated circuits/lines |
|||||||
External Port |
Internal Port |
|||||||
Coupling mode: Line-to-line |
Coupling mode: Line-to-earth |
Coupling mode: Line-to-line |
Coupling mode: Line-to-earth |
|||||
Voltage |
Current |
Voltage |
Current |
Voltage |
Current |
Voltage |
Current |
|
0 |
NA |
NA |
NA |
NA |
NA |
NA |
NA |
NA |
1 |
NA |
NA |
NA |
NA |
NA |
NA |
0.5V |
12A |
2 |
NA |
NA |
NA |
NA |
NA |
NA |
1kV |
24A |
3 |
NA |
NA |
NA |
NA |
NA |
NA |
2kV |
48A |
4 |
NA |
NA |
4kV |
95A |
NA |
NA |
4kV |
95A |
5 |
NA |
NA |
4kV |
95A |
NA |
NA |
4kV |
95A |
Tables 3a and 3b: IEC 61000-4-5 - selection of test levels on signal lines per installation classes
Shielding the Interfaces of Surveillance Cameras with Transient Suppression Devices
This blog initially addressed the increasing prevalence of outdoor surveillance camera usage. Having thoroughly explored surge events and the IEC 61000-4-5 standard for signal lines, let's examine methods to safeguard the ports and interfaces of a surveillance camera from surges and other EOS events.
The typical suppression element required, as per the IEC 61000-4-5 standard, is a clamping device placed in parallel with the circuit that needs protection. In power supply or VBus applications, high-power devices are often necessary. Depending on the specific application and the position of the component in the circuit board (whether at the input or middle of the board), one might need either an individual discrete device or an assembled solution. Transient Voltage Suppression (TVS) diodes stand out due to their exceptional clamping voltage characteristics and rapid response time for safeguarding data or signal lines and providing secondary board-level protection. The objective of any transient suppression device is to endure the surge current and protect the electrical circuit by constraining the surge voltage to a level below the circuit's maximum allowable voltage.
The TVS diodes respond promptly to voltage surges, clamping the surge to a specified value before entering the circuit— clamping voltage (VCL). When a transient event occurs, the TVS diode transforms into a low-impedance device, redirecting the transient surge current away from the circuit and providing essential protection. The surge current capability is specified as peak pulse current (IPP) in the datasheets of the TVS diodes.
Figure 5. Block diagram of a surveillance camera and its interfaces
Figure 5 shows a typical surveillance camera's block diagram with interfaces that need protection from electrical overstress events, including ESDs and surges. Table 4 lists the transient protection devices to shield the ports and interfaces of a surveillance camera, sensors, servers, and other devices from electrical transients, electrical overstress (EOS) and ESD events (Figure 5).
Interface to Protect |
Part Number |
# of Lines |
Configuration |
VRWM |
ESD Rating Contact/Air |
Surge Rating (8/20µs) |
Clamping Voltage (8/20µs) |
Cap (Max) |
Package Dimensions |
アンテナ |
1 |
Bidirectional |
22V |
±25kV/±30kV |
18A |
15V |
0.5pF |
DFN (1.0x0.6x0.55mm) |
|
Speaker |
1 |
Bidirectional |
5V |
±30kV/±30kV |
7.5A |
9.2V |
15pF |
DFN (1.0x0.6x0.55mm) |
|
Memory |
1 |
Bidirectional |
5V |
±20kV/±30kV |
6A |
6.5V |
7pF |
DFN (1.0x0.6x0.55mm) |
|
HD-HDI |
1 |
Bidirectional |
3.3V |
±8kV/±15kV |
7A |
5.3V |
0.25pF |
DFN (1.0x0.6x0.55mm) |
|
Side button |
1 |
Bidirectional |
5V |
±20kV/±30kV |
6A |
6.5V |
7pF |
DFN (1.0x0.6x0.55mm) |
|
センサー |
1 |
Bidirectional |
3.3V |
±10kV/±17kV |
9A |
5.4V |
0.25pF |
DFN (0.6x0.3x0.25mm) |
|
RS-485 |
2 |
Bidirectional |
12V |
±15kV/±20kV |
100A |
40V |
12pF |
DFN (1.6x1.6x0.55mm) |
|
USB 2.0 |
1 |
Bidirectional |
4.0V |
±30kV/±30kV |
20A |
8V |
0.65pF |
DFN (1.0x0.6x0.55mm) |
|
PoE |
1 |
Unidirectional |
58V |
±15kV/±20kV |
20A |
70.2V |
72.8pF |
DFN (1.6x1.6x0.55mm) |
|
イーサネット |
4 |
Bidirectional |
3.3V |
±30kV/±30kV |
40A |
25V |
5pF |
DFN (3.0x2.0x0.6mm) |
Table 4: ESD and surge protection devices for surveillance cameras per interfaces and ports
Conclusion
Power over Ethernet (PoE), simplified installation, and enhanced data communication with portable devices have collectively contributed to the widespread adoption of surveillance cameras in various outdoor locations. Given the extensive use of this device, it becomes imperative to ensure robust protection for every interface, data connection, and communication port against ESD and surge-related EOS threats reliably over the installation's lifetime. In most instances, a TVS diode offers dependable protection over an extended period. Nevertheless, due to outdoor surveillance cameras' exposure and associated cables to lightning and switching surge hazards, a meticulous choice of transient suppression devices becomes essential. Semtech, a prominent manufacturer of EOS protection products, adheres to IEC 61000-4-5 standards for surge protection and IEC 61000-4-2 standards for electrostatic discharge (ESD) in the signal lines of surveillance camera designs. Our trusted TVS products have been the choice for many popular surveillance cameras worldwide. Contact Semtech while designing your circuit for a surveillance camera and protect it from EOS events in the data/signal lines.
Semtech®, the Semtech logo, RClamp®, TClamp®, and µClamp® are registered trademarks or service marks of Semtech Corporation or its affiliates. Other product or service names mentioned herein may be the trademarks of their respective owners.